Waste is a failure of the imagination
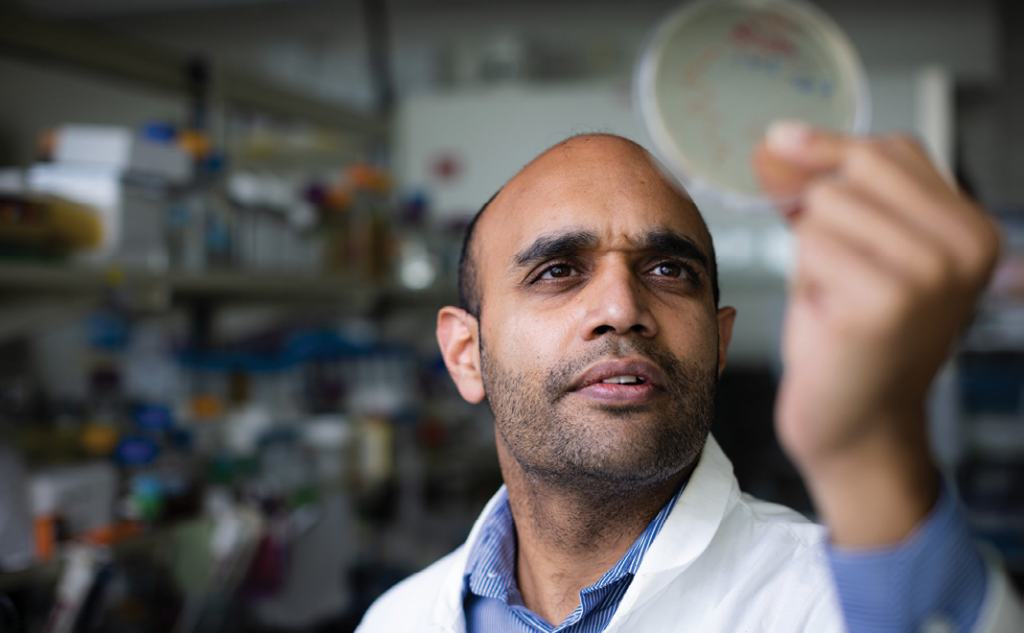
The atmosphere contains 2,200 gigatonnes of carbon dioxide (CO2). Each year, 58 gigatonnes from this reservoir are sequestered by earth’s processes. At the same time, 63 gigatonnes of CO2 are emitted by all human activities and natural phenomena. For much of the planet’s existence, the rates of emission and sequestration of CO2 have been balanced. The current push toward net-zero is an attempt to reset the balance. Humanity has no future if we fail to do so.
Internal combustion engine (ICE) vehicles cumulatively emit approximately 6 gigatonnes of CO2 each year. Widespread replacement of gasoline guzzlers with electric vehicles (EVs) could reduce global CO2 emissions by 3 gigatonnes. That is 60% of our 5 gigatonnes target! The production of more EVs means more mining.
Then, what is the issue? Several.
For starters, new mines are increasingly difficult to find, permit, and develop. Declining ore grades means they are less productive and are more expensive to operate. We desperately need larger, more enriched sources of metals. More mining also means more waste rock and more tailings ponds. The current global inventory of tailings exceeds 200 billion tonnes and 200 trillion litres of water reside in tailings ponds. In many cases, mining waste directly contributes to the generation of acid rock drainage (ARD), which is a significant environmental challenge with a particularly high carbon penalty. Tailings and ARD are also leading contributors to the mining industry’s attached liabilities.
What if I were to tell you that one of the problems is a solution to the other? Consider the example of copper: The global reserves of copper are estimated to be 880 million tonnes. A quarter of these reserves – 200 million tonnes – reside in Chile. In fact, every report cites Chile as holding the largest reserves of copper in the world. It is a contentious claim. Did you know that 200 million tonnes of copper also sit in tailings around the world, a vast majority of which are in acidic ponds? Imagine if one were to mine these waste sources rather than expend significant resources to store or neutralize them. There now exists a net-zero technology that can extract valuable metals from the water in tailings ponds and tailings reprocessing facilities and simultaneously treat and condition the water. It is called TersaClean, and field testing of the technology is expected to commence next summer.
TersaClean is the brainchild of Tersa Earth Innovations, a biotechnology company that is developing decarbonized processes for metal recovery and water treatment solutions for the mining industry. Tersa has developed technologies for ARD treatment, direct lithium extraction, and even PFAS (polyfluoroalkyl substances) remediation.
TersaClean combines three distinct goals: metal recovery, water treatment, and carbon abatement, into a single process. TersaClean is a two-stage, continuous process. The first stage comprises a stack of flow-based microbial fuel cells (MFCs) that selectively recover metals from mine-impacted water. The water is further processed in the second stage using microbial carbonate precipitation (MCP) and conditioned to a state that meets environmental regulations for discharge and recycle.
MFC is a self-powered electrochemical cell – a bacteria-driven battery – that consists of cathodic (reducing) and anodic (oxidizing) chambers sandwiching an ion exchange membrane. Natural or engineered exoelectrogenic (electron generating) bacteria at the anode generate an electric current through the oxidation of an organic substrate such as glucose, acetate, or lactate. The current is relayed to the cathode, where it is consumed in reduction reactions that deposit metals in solution onto the cathode. The phase of the deposited metal is influenced by the pH and temperature of the solution and is guided by Pourbaix diagrams. Metals such as gold, silver, iridium, platinum, and palladium are deposited in their pure, elemental forms. Copper deposits in the pure form under acidic pHs. Contaminants such as arsenic deposit in the oxidized form, whereas selenium is recovered in its elemental form under typical conditions. MFCs operate on the simple premise of allowing bacteria to do what they have evolved to do best – oxidize sugars and respire. In the case of exoelectrogenic bacteria, the respiratory products are electrons. This fact, along with separation of the redox couple, delivers higher rates that are independent of concentration of the metals in solution. Flow-based MFCs drive this advantage several steps forward.
The rate of recovery of the metals is influenced by the rate at which water flows through the cathodic chamber of the MFC, the metabolic rate of the exoelectrogenic bacteria, and the electrode potentials. The company has made several innovations ranging from materials chemistry to improve electrode performance to strain optimization and process engineering that facilitate precise control over critical operating parameters. The continuous flow of water through the chamber also generates ideal mixing conditions, which ensures that the system is rarely limited by mass transport. The ability to impose a voltage gradient across the cathode also allows metals to be separated individually at source. Significantly, the MFCs can be combined in series and parallel within a stack, which expands treatment options for mining companies.
Following the removal of metals and initial de-acidification, water is then completely neutralized by the addition of a carbonate salt in the second stage. The resulting mixture is then subjected to a second biotreatment, wherein MCP regenerates the carbonate salt that was added previously. MCP employs engineered, ureolytic strains of bacteria that convert urea to carbonate anions. The carbonate salt crystals nucleate and accumulate on the cell surface and are subsequently used for continuous neutralization of the acidic stream in a closed loop. Like the MFCs, the rate of conditioning of the water by the MCP step too can be increased by varying the metabolic rate of the microorganism and the volumetric flowrate.
Tersa’s deployment scheme consists of integrating the MFC stack, the MCP reactor, and associated fluid handling operations onto an environmentally insulated shipping container. The shipping containers will serve as mobile process plants when deployed at an active mine or remediation site. Rate enhancements can be achieved by controlling a range of parameters, including volumetric flow rate, number of MFCs in the stack, metabolic rates of the microorganisms, electrode potentials and the magnesium carbonate recycle rate in the closed loop MCP step. Volumetric scalability can be achieved by increasing the number of shipping containers.
TersaClean has been validated in the laboratory. It achieves high rates and percentages of metal removal and outputs water that meets regulatory requirements. The readiness level of the process is nearing six.
The design and operation of TersaClean is fundamentally detached from the scaling laws that limit conventional batch-based wastewater treatment operations. Batch processes have low intrinsic rates of treatment that are challenging to modify. Therefore, the only way to scale a batch-based treatment solution is to increase the volume of the operation. The relationship between capital/operating expenditures and volume is linear, implying that there are limited economies of scale. In contrast, TersaClean perform and cost the same at all scales.
This technology is challenging the conventional notion that mining must generate waste and shifting the paradigm to view tailings as ore. Tersa’s vision is to re-cast mining from being a linear exercise that reshapes landscapes to one that is circular and reclaims the earth.
More broadly, small communities and First Nations have constantly endured the most of haphazard industrialization. Tersa is working directly with and in these communities to remediate their lands and benefit from the economic windfall that our technology delivers.
Vikramaditya Yadav is an associate professor at the department of chemical & biological engineering and the school of biomedical engineering, director of the Master of Engineering Leadership in sustainable process engineering at the University of British Columbia, and CEO of Tersa Earth Innovations.
Comments